Synesthesia: crossed wires or free association?
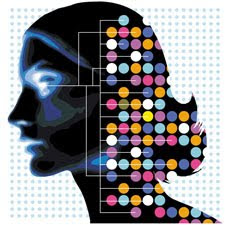
For millennia, philosophers have mused over the nature of perception, how closely it mirrors “reality” and whether different people might, quite without knowing it, subjectively perceive the world in very different ways. We might agree that an apple is “red”, but is the quality of your experience of its redness the same as mine? This has seemed an almost impossible nut to crack, but the fascinating condition of synesthesia provides a stark example where the quality of subjective experience is very definitively and demonstrably different. This may be due to genetic variants which affect the functional segregation of specialized circuits in the brain which makes synesthesia even more interesting. The following is a book review I recently wrote for the American Journal of Human Biology, of: Wednesday is Indigo Blue: Discovering the Brain of Synesthesia . By Richard E. Cytowic and David Eagleman. X + 309 pp. Cambridge, MA: MIT Press. 2009. For people with synesthesia, parti