Schema formation in synaesthesia
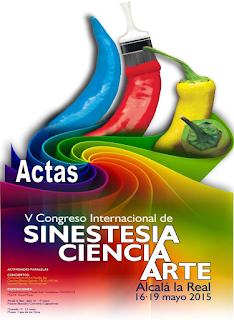
Many of the ideas were also developed in a paper with my colleague Fiona Newell, on Multisensory Integration and Cross-Modal Learning in Synaesthesia: a Unifying Model.
Abstract
Psychologists use the term “schema” to
refer to the information or knowledge that makes up our concept of an object. It
includes all the attributes that the object has, such as the shapes of a letter
and the sounds it can make, the shape of a numeral and the value it represents,
or the face of a person and their name and everything you know about them. In
many cases, those attributes are represented across very different brain areas
(such as those conveying visual or auditory information, for example). With
experience, the representations of the different attributes of an object become
linked together, in the mind, by repeated co-occurrence.
At the level of the brain, this must
involve some kind of strengthening of connections across areas of the cerebral
cortex so that a pattern of activity in one area (say that induced by the sight
of the letter “A”) reliably co-activates or primes a particular pattern of
activity in another area (say the sounds of the letter “A”). The brain is wired
to enable this kind of communication between different areas, so that these
sorts of associations can be learned by repeated exposure to contingent stimuli,
for example as we learn the alphabet or our numbers.
Synaesthesia is characterised by the
incorporation of additional attributes into the schema of an object – ones not
reflecting the characteristics of the object itself but some internal
associations triggered by it. I propose a model to account for synaesthesia
based on innate differences in wiring between cortical areas, which lead to
additional percepts (such as colours) being triggered by an object during
learning. These repeated patterns eventually result in stable synaesthetic
associations, despite the lack of reinforcement from the external world. This
model can account for the heritability of the condition and evidence of
cortical hyperconnectivity, but also for the learned nature of many of the
inducing stimuli and observed trends in letter-colour or word-taste pairings.
In this conceptual framework, a natural
contrast emerges between synaesthesia, on the one hand, and a number of other conditions,
collectively known as “agnosias”, on the other. These include dyslexia and
dyscalculia, face blindness, tone deafness, colour agnosia and others. These
conditions seem to reflect an inability to incorporate all the attributes of an
object into a schema, resulting in a “lack of knowledge” of particular types of
objects. There is evidence that these result from decreased connectivity
between brain regions. It is hoped that the study of synaesthesia may also
inform on the mechanisms underlying these less benign conditions.
Introduction
Synaesthesia is often
described as a cross-sensory phenomenon, where, for example, particular sounds
(such as words or musical notes) will induce a secondary percept (such as a
color or taste), which is specific for each stimulus (Bargary and Mitchell, 2008; Hubbard and Ramachandran, 2005). While these florid types of synaesthesia
involve very vivid perceptual experiences, the more common manifestation is
associative (Simner, 2012). These cases involve the certain knowledge
that some object, such as a letter or number, has, in addition to its normal
attributes (shape, sound, value, etc.), some extra traits associated with it,
such as spatial position, color, texture, even gender and personality. These associated characteristics are stable,
idiosyncratic and have typically formed an intrinsic part of the person’s
schema of that object for as long as they can remember.
A recurrent question in relation to
synaesthesia is whether it represents a truly distinct phenomenon,
qualitatively different from typical perception, or reflects instead an
amplification or exaggeration of normal processes of multisensory integration (Deroy and Spence, 2013b; Ward et al., 2006). A
related question concerns the extent to which particular synaesthetic
associations arise arbitrarily through intrinsic neural mechanisms or are
driven instead by experience and learning in ways that may be common to all
people (Watson et al., 2014). Both these questions bear on what is arguably the central question
in the field: why do some people develop synaesthesia while most do not? The
answers to these questions thus determine fundamentally how we conceive of
synaesthesia.
On the one hand, synaesthesia represents a
dichotomous phenotype – people are relatively easily categorised as
synaesthetes or non-synaesthetes. Moreover, the condition is clearly genetic in
origin, often running in families with a Mendelian pattern of inheritance (some
members clearly having the condition, others clearly not) (Asher et al., 2009; Barnett et al., 2008; Baron-Cohen et al., 1996; Galton, 1883; Rich et al., 2005; Ward and Simner,
2005). The
primary answer to the question of why some people develop synaesthesia is therefore
that they inherit a genetic variant that strongly predisposes to the condition.
This argues for some intrinsic difference as a necessary starting point in
explaining the condition and against a model where general processes are
sufficient to explain it.
On the other hand, a number of lines of
evidence suggest that whatever is happening in synaesthesia, it relies on or at
least interacts with processes of multisensory integration that are common
across all people. These include both acute cross-sensory activation as well as
longer-term cross-modal learning.
First, there is strong evidence that most
areas of what has been deemed unisensory cortex are in fact essentially
multisensory, with extensive anatomical cross-connectivity and at least some
modulatory inputs from other modalities providing credible substrates for
cross-sensory interactions (Ghazanfar and Schroeder, 2006; Qin and Yu, 2013). The
idea that such interactions may be always present but not always consciously
accessible is reinforced by the activation of visual areas in blind or
blindfolded people (Bavelier and Neville, 2002) and by the phantasmagoric audiovisual synaesthetic experiences associated
with certain hallucinogens, such as lysergic acid (LSD), psilocybin or
mescaline (Schmid et al., 2014; Sinke et al., 2012). Such
experiences indicate that the barriers between the senses are certainly not as
rigid as typical experience suggests.
However, drug-induced synaesthetic
experiences have quite a different phenomenology, tending to involve florid,
detailed and complex visual experiences induced by sound, especially music (Deroy and Spence, 2013b; Sinke et al., 2012). By
contrast, developmental synaesthesia is characterised by more stable and sedate
cross-sensory pairings of particular stimuli with particular additional
percepts or conceptual attributes. These tend to be quite simple in nature,
involving perceptual primitives rather than complex forms. The relevance of
drug-induced synaesthesia to the mechanisms underlying developmental forms thus
remains unproven.
There is another line of evidence, however,
which supports the idea that normal multisensory integration processes are
involved in synaesthesia. In particular, these are processes involved in
categorical perception, which integrate information about objects across
sensory domains and generate conceptual and supramodal representations.
For any form of synaesthesia, the
particular pairings that emerge between inducers and concurrents are
idiosyncratic and tend to be dominated by apparent arbitrariness in any
individual. However, by looking across many synaesthetes, it is possible to
discern clear trends in such pairings, for example between particular letters
and their synaesthetic colours. In English speakers, the letter B may be more
commonly blue than other colours (perhaps 30% of the time) and the letter Y
more commonly yellow (as high as 50% of the time) (Barnett et al., 2008; Rich et al., 2005). It
is even apparent that, for some synaesthetes, all of their colour-letter
pairings are derived from experience with childhood toys, such as refrigerator
magnets (Witthoft and Winawer, 2006). Similarly, for many synaesthetes with number forms, the numbers 1
to 12 are arranged in a circle like a clock face (Galton, 1883). Many word-taste pairings can also be explained by semantic
associations, such as “Cincinnati” tasting of cinnamon and “Barbara” tasting of
rhubarb (Simner, 2007).
There are thus clear cultural and semantic
influences on the particular pairings that emerge in developmental
synaesthesia. Some theorists have argued that such trends demonstrate that
synaesthesia is induced by learning and, even further, that the purpose of synaesthesia is to aid in
learning the inducing categories (Asano and Yokosawa, 2013; Mroczko-Wasowicz and
Nikolic, 2014; Watson et al., 2012; Yon and Press, 2014).
How can the idea that synaesthesia reflects
innate, genetic differences be reconciled with models that suggest it is driven
by learning? Here I develop a theoretical framework showing that these two
models are quite compatible (previously sketched out in (Mitchell, 2013)). I argue: (i) that the predisposition to develop synaesthesia at
all is genetic and innate; (ii) that the particular form and the pairings that
emerge are driven largely by idiosyncratic connectivity differences; but (iii)
that because the processes through which such pairings consolidate over time
involve normal mechanisms of multisensory learning and categorical perception,
the outcome can also be influenced by experience.
Synaesthesia
as an associative phenomenon
Developmental synaesthesia can be present
in diverse forms and experienced in qualitatively distinct ways. One important
distinction is between synaesthetes who are “projectors” and those who are
“associators”. For the former, the concurrent percept is actively and vividly
perceived, either out in the world or “in the mind’s eye”, while for the latter
it is merely conceptually activated in the way that saying the word “banana”
activates the concept of yellow, possibly even prompting a visual image of the
object in that colour, but is unlikely to induce a veridical percept of yellow
out in the world.
There is another important phenomenological
distinction between lower-level, truly cross-sensory synaesthesia, and
higher-level, more conceptual forms. In the former, taking coloured hearing as
an example, any sound may induce a visual percept, whether the person has ever
heard it before or not. The same sound will tend to induce the same visual
percept, but this does not seem to require prior experience.
For many associative forms, however, the
synaesthetic associations arise only with a particular set of stimuli.
Crucially, these are almost exclusively stimuli that are (i) categorical, and
(ii) learned (often over-learned), such as letters, numbers, days of the week,
months of the year, musical notes, words, etc. The emergence of these
associative forms of synaesthesia must thus necessarily involve learning at some
level, and indeed clearly interacts with normal processes through which the multisensory
attributes of objects are learned.
Cross-modal
learning and categorical perception
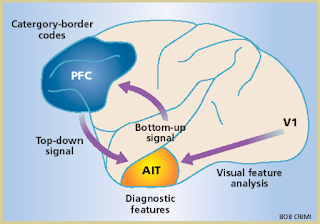
Even for recent inventions like written
alphabets, these areas tend to develop in the same positions across people (Dehaene and Cohen, 2007). This suggests that the specialisation of cortical areas for
particular classes of objects relies on an evolutionarily programmed pattern of
connectivity that places them at a convergence point of multiple, parallel
hierarchies, enabling them to integrate information across the relevant sensory
modalities.
Such areas are differentially activated by
tasks that tap into semantic knowledge and lesions or temporary inactivation of
these areas can result in object agnosias (De Renzi, 2000) – the inability to access all the attributes of an object when the
concept is activated (Chiou et al., 2014; Kourtzi and Connor,
2011; Mitchell, 2011). For
example, prosopagnosia, or face blindness, represents an inability to recognise
people’s identity from their faces, though other aspects of face processing may
remain intact. Similarly, colour agnosia refers to the inability to link
characteristic colours into the schemas of objects, despite normal colour
perception and discrimination.
Both these conditions can be caused by
injuries to associative areas, but, intriguingly, both can also be innate and
inherited (Behrmann and Avidan, 2005; Duchaine et al., 2007; Nijboer et al., 2007; van Zandvoort et al., 2007). The
fact that such conditions can be genetic highlights the fact that differences
in brain wiring (either structural or functional) can impact, very selectively,
on higher-order conceptual processes, presumably by altering the anatomical
substrates through which perceptual information is processed (Mitchell, 2011). Indeed, structural and functional neuroimaging studies have
highlighted reduced connectivity within extended networks of cortical areas in
these conditions. By contrast, synaesthesia may be caused by hyperconnectivity,
linking additional areas into the conceptual schemas of learned categories of
objects (Mitchell, 2011).
The processes by which such schemas emerge
can be illustrated by considering how letters are learned. As children are
learning to read they must learn to recognise and distinguish the various
graphemes of the alphabet and also link them to the appropriate phonemes of
their native language (Blomert and Froyen, 2010). In the visual domain, recognising graphemes requires, firstly,
extraction of increasingly complex visual features across the hierarchy of
areas in the ventral visual stream (Kravitz et al., 2013). Though it is a simplification, it is roughly true that each level in
the visual hierarchy extracts more complex features by integrating inputs from
multiple neurons at the level below, eventually enabling representation of
shapes and objects across the visual field.
Areas that are higher still become
specialised for processing specific types of visual information that correspond
to various categories (letters, faces, objects, scenes). This kind of
categorical perception enables the recognition of various instances of an
object – different sizes, views or versions (such as of the letter “A” (A,
A, A, a)) – all of which can activate the representation of the concept of
the object (Kravitz et al., 2013). Similar processes arise as children learn spoken language – they
develop expertise in recognising the typical speech sounds of their native
language, but become deficient in distinguishing between uncommonly used
phonemes.
Neuronal networks in general can learn in
the following fashion. Any given stimulus will activate a distinct subset of
neurons across an area of cortex, which can be thought of, reasonably
accurately, as a two-dimensional sheet of highly interconnected cells. Due to
their coincident activation, the connections between these neurons will be
slightly strengthened (Hebb, 1949). If a particular stimulus is seen over and over again, this subset
of neurons will become a functional unit, primed to respond en masse to similar
stimuli. (More realistically, the properties of the stimulus may be represented
not by one static pattern but by the dynamic trajectory of firing patterns across
some time period (Daelli and Treves, 2010)).
The patterns of neuronal activity that
represent any one object can be thought of as attractor states – any stimulus
that occupies a nearby spot in perceptual space (e.g., that has a similar shape
or sound) will be “pulled into the attractor”, with the network state
ultimately converging on a pattern that represents that object. This
“perceptual magnet” effect can be observed in psychophysical results, which
show that discrimination between stimuli that fall within a boundary is less
than that between two stimuli that are equally distant in stimulus parameters
but that span a categorical boundary (Daelli and Treves, 2010). It is important to note that this perceptual categorisation,
especially of ambiguous stimuli, is also sensitive to context and top-down
influences (Feldman et al., 2009) – indeed, all perception
involves the comparison of bottom-up signals with top-down expectations, or
prior probabilities, so as to allow active inference of the objects in the
world that are responsible for the pattern of sensory stimulation (Friston, 2010; Gilbert and Li, 2013).
Linking the visual and auditory attributes
of an object requires yet a higher level of integration and abstraction (Kourtzi and Connor, 2011). It is driven by the statistical regularities of experience – when the
letter A is seen, it is typically accompanied by the sound ā, as in hay, or ă, as in cat. The representations of these
shapes and sounds are thus reliably contingent and can lead to the development
of a higher-level representation, incorporating both elements. The brain thus builds up cognitive representations of letters as
audiovisual objects with characteristic attributes, despite the fact that these
are essentially arbitrary pairings between symbols and sounds, determined
purely by convention (Blomert and Froyen, 2010).
These processes reinforce each other. Though
spoken language is learned much earlier and much more easily than written
language, learning to read nevertheless increases the ability to distinguish
between phonemes (which are not the natural basic units of speech) (Blomert and Froyen, 2010; Dehaene et al., 2010).
Conversely, phonetic representations are involved in strengthening categorical
representations of graphemes (Brem et al., 2010). The
process of forming these associations is quite protracted, taking years to
reach a level of perceptual expertise that is effectively automatic, as
demonstrated by cross-modal mismatch negativity signals (Blomert, 2010). The development of such automaticity is lacking in dyslexia,
presumably contributing to the fact that reading remains effortful despite
extensive training.
In this model of schema formation, any areas
that are reliably co-activated will be incorporated into the schema of an
object, as supramodal areas monitor patterns of co-activation across many lower
areas and represent the statistical regularities of such contingencies. This
leads to a model of synaesthesia whereby innate differences in brain wiring
produce internally generated percepts, which, over time, are incorporated
through the normal processes of cross-modal learning into the schemas of the
inducing objects.
A
model unifying innate differences with learning processes
This model of associative synaesthesia
requires in the first place some intrinsic cross-activation of additional areas
not normally activated by particular objects (an innate difference between
synaesthetes and non-synaesthetes). For letters, this might be an area
representing colour, for example (but the idea can be readily extended to other
forms). If such an area is topographically interconnected with say the grapheme
area, so that nearby neurons in the first area project to nearby neurons in the
second area (Bargary and Mitchell, 2008), then activation of the pattern of neuronal firing that represents
any particular letter will necessarily cross-activate some (arbitrary) pattern
of neuronal firing in the colour area (Brouwer and Heeger, 2009; Li et al., 2014).
Given that the colour areas mature much
earlier than the grapheme area (Batardiere et al., 2002; Bourne and Rosa, 2006; Dehaene and Cohen, 2007), such
patterns will likely evolve towards a set of attractor states that already
represent specific colours (Brouwer and Heeger, 2009; Li et al., 2014). This
may or may not lead to a conscious and vivid percept of colour, but should at
least lead to the activation of the concept of a colour. Over time, with
extensive repetition, this internally generated sensory property will come to
be incorporated into the schema of that letter, becoming as much a part of the
concept of the letter as its shape(s) and sound(s).
In this model, without any other
influences, the particular pairings that emerge would be expected to be largely
arbitrary – dependent on the particular cross-connectivity at the anatomical
level. Such a model could explain observed second-order trends, whereby
similarly shaped letters tend to have similar colours within individual
synaesthetes, even though these colours differ across synaesthetes (Watson et al., 2012). The letters E and F, for example, are often similarly coloured,
which would be expected from an arbitrary topographic mapping between areas
representing shape space and colour space (Brang et al., 2011).
In addition, one can imagine how the
pairings might be influenced by characteristics that affect the types of neural patterns representing
specific letters or colours (Chiou and Rich, 2014; Deroy and Spence,
2013a; Ward et al., 2006; Watson et al., 2012). For
example, there could be some correspondences between the states representing
higher frequency letters and those representing higher intensity colours (such
as the proportion of neurons within the area that are recruited to the
representational pattern or some dynamical property of the pattern), which
would make pairings between members of those two types more likely to emerge. While
speculative, this kind of scenario may provide an explanation of cross-sensory
trends that do not rely on semantic information but reflect some currently
unknown representational parameters that hold across modalities.
As with the pairings between graphemes and
phonemes that emerge during learning to read, synaesthetic pairings also take a
long to coalesce. Simner and colleagues found in a longitudinal study of
children an increase in both the number and stability of synaesthetic
correspondences between letters and colours at age 7/8 compared to age 6/7 (Simner et al., 2009) and more again at age 10/11 (Simner and Bain, 2013).
Importantly for this model, this protracted
period of consolidation leaves the opportunity for semantic associations to
influence the ultimate outcome, in the same way that they do when learning the
attributes of any object. Such influences could explain the observed trends in
specific pairings mentioned above. For example, when a synaesthete is learning
the letter B, and their colour area is being cross-activated in some arbitrary
pattern, the semantic relationship between B and “blue” may prime the neuronal
pattern representing blue, making it more likely for the network to move toward
that attractor state, which will in turn be reinforced by each such
co-activation. This can be interpreted in a Bayesian context as top-down
signals conveying a prior probability of “blue”, in the context of the letter
B, which, to a greater or lesser extent across individuals, will tend to
over-ride the bottom-up sensory information, biasing the incorporation of a
consistent association with this colour into the emerging schema of the letter.
It is not difficult to see how such semantic influences could act in other
kinds of synaesthesia – for example, if the numbers 1 to 12 are regularly seen
in clock face arrangement, that may influence the spatial pattern that emerges
in an individual’s number line. (It will be interesting to see whether this
trend changes as clock faces become rarer).
In this way, the particular synaesthetic
associations that emerge through this protracted process can be biased by
top-down semantic processes, without being entirely determined by them,
reflecting observed trends rather than rules of associations. Fundamentally,
this means that while such semantic processes may affect the outcome, they are
not the prime drivers of the phenomenon of synaesthesia. The condition of
synaesthesia is thus not caused by
learning, and there is certainly no reason to think of it as having a purpose in facilitating learning. It may
have that effect, but it is a conceptual mistake to interpret that as a reason
for its existence.
The difference that makes a difference, in
determining why some people get synaesthesia and others do not, is genetic
(clearly so in many cases and likely so in others). The model of
cross-activation at some level of the perceptual hierarchy remains a
parsimonious one for the mechanism through which these genetic differences
mediate their primary effects (Bargary and Mitchell, 2008; Hubbard et al., 2011), and
has at least general support from many neuroimaging studies (Rouw et al., 2011). Whether this involves primary changes in structural or functional
connectivity remains an open question, but in either case, the outcome is an
altered sensory experience, with some internally generated percept that gets
assimilated into the schemas of the inducing objects through normal processes
of multisensory learning.
This model is subtly but importantly
different from ones that explain synaesthesia on the basis of only an acute
difference between the brains of synaesthetes and non-synaesthetes. For some
synaesthetes, such as audiovisual synaesthetes for whom new sounds induce a
vivid visual percept, there may – perhaps must – be some ongoing sensory
cross-talk. But for associators, their synaesthetic experiences may arise not
because their brain wiring is
slightly different, but because that difference existed over development, while
the person was learning various categories of objects. An interesting
possibility that may link the phenomenologically distinct forms of projector
and associator synaesthesia is that an early state of vivid synaesthesia, which
may fade in conscious experience over time (as reported at least anecdotally by
some adults), could nevertheless lead to long-lasting conceptual associations
if it were present during this learning period.
The theoretical framework presented here is
consistent with both an innate difference as the fundamental driver of the
condition of synaesthesia, and with semantic and experiential influences on the
eventual phenotype that emerges. In particular, it proposes that the internally
generated synaesthetic percepts are treated similar to other sensory
information as the brain is learning the sensory attributes of objects and
developing schemas to conceptually link them.
References
This is great information explaining how the brain is working. Are there any researchers in the practical application for teachers and parents? I have yet to find anyone that can help with my 13 -year-old.
ReplyDelete